視覚前野
伊藤 南
東京医科歯科大学生体機能システム学分野
DOI:10.14931/bsd.3705 原稿受付日:2013年5月24日 原稿完成日:2021年9月22日
担当編集委員:田中 啓治(国立研究開発法人理化学研究所 脳神経科学研究センター)
英語名:extrastriate cortex、circumstriate cortex 独:extrastriärer Kortex 仏:cortex extrastrié
同義語:外線条皮質、有線外皮質、後頭連合野
視覚前野は哺乳類の大脳新皮質の一部で、後頭葉の視覚連合野(後頭連合野)、ブロードマンの脳地図の18野、19野に相当する。V2、V3、V3A、V4、V5/MT、V6等の機能的領野に区分される。第一次視覚野(V1、17野)より主な入力を受けて視覚情報処理を行う。各領野のニューロンは受容野を持ち、レチノトピー(網膜部位再現)の性質を示して、片半球の領野が反対側の半視野を表す。これらの領野は階層的な結合関係を持ち、上の階層の領野ほど受容野が大きく、より複雑な刺激特徴の情報を抽出表現する。主に2つの視覚経路に分かれており、腹側視覚路はV2、V4を介して側頭葉(側頭連合野)に出力し、物体の形状や物体表面の性質(明るさ、色、模様)を表し、視覚対象の認識や形状の表象に寄与する。背側視覚路はV2、V3、V5/MT、V6を介して後頭頂葉(頭頂連合野)に出力し、3次元的な空間配置、空間の構造、動きを表して、眼や腕の運動制御に寄与する。
視覚前野とは
哺乳類の大脳新皮質の一部で、後頭葉の視覚連合野(後頭連合野)、あるいは後頭葉から第一次視覚野(V1)を除いた部分。細胞構築学的にはブロードマンの脳地図の18野、19野に相当する。18野を前有線皮質(傍有線野、prestriate cortex)、19野を周有線皮質(周線条野、後頭眼野、parastriate cortex)、視覚前野全体を外線条皮質(有線外皮質、extrastriate cortex、circumstriate cortex)と呼ぶ。当初、第一次視覚野(V1)に隣接する領域を広く視覚前野ないし視覚連合野と称した。1960年代以降、単一細胞記録やトレーサーの注入による研究が進み、ニューロンの応答特性、受容野の大きさや位置、解剖学的投射などを手がかりとした機能的領野区分の研究がネコやサルで盛んになった。また免疫組織化学による研究が進み、タンパク質や遺伝子の発現に着目した研究も進んだ。1980年代以降、fMRIや光計測等のイメージング技術の発達により視野地図の広がりを可視化する研究が盛んになり、ヒトを対象とする研究も進んだ。機能的な領野区分は旧世界ザルのマカカ属サル(アカゲザル、ニホンザルなど)で最も進んでおり、V2、V3、V4、V5/MT、V6等の機能的な領野が同定され、それぞれが個別の領野として扱われることが多い。細部や高次領域(V3、V4、V6)については、ヒトを含む動物種により区分法や名称が異なり、研究者間でも見解の相違がある。本稿では旧世界ザルの知見を中心に概説する。
機能的な領野の区分
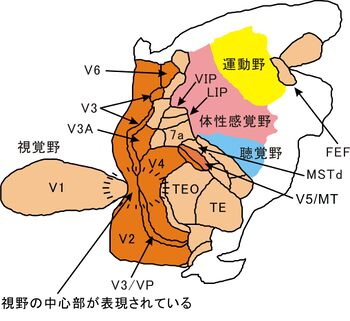
大脳皮質の表面をのばして表示したもので、内側で切って上下に開いたように表示してある。右側が前頭葉(前側)、左側が後頭葉(後側)、橙色の部分が視覚前野、肌色がその他の視覚野を示す。(Felleman and Van Essen (1991)[1] Fig.2を改変)
視覚前野のニューロンは、V1と同様に、(古典的)受容野内に呈示された視覚刺激が持つ刺激特性を抽出する。視覚刺激の位置情報は受容野の位置で表される。視覚前野の各領野はレチノトピー(網膜部位の再現)の性質を示し(詳細は受容野を参照)、片半球の1つの領野が反対側の視野を映す一枚のトポグラフィックな視野地図を表す。受容野の位置が中心視野(fovea)から周辺視野に移るにつれて、受容野の大きさは大きくなる。領野内で中心視野を表す部分の面積は大きく、周辺視野に移るにつれて占有面積の割合が減少する(V6は除く)。マカカ属サルのV2、V3、V4はそれぞれV1の前方に帯状に広がり、大脳皮質の腹側の領域が反対側の視野の上半分(上視野)を表し、背側の領域が視野の下半分(下視野)を表し、その間の領域が中心視野を表す。領野の境界は視野の垂直子午線(vertical meridian)ないし水平子午線(horizontal meridian)を表す。垂直子午線付近のニューロンは脳梁を介して反対側の半球から入力を受け、両側の視野にまたがる受容野を持つ。V1、V2、V3、V4の中心視野領域は月状溝(lunate sulcus)の終端部付近に収束している。この付近では受容野が小さくその差違が明瞭でないので、領野の境界を正確に定めることが難しい。V3、V4の区分には諸説がある(後述。V3,V4の項を参照)。V5/MTは上側頭溝(superior temporal sulcus、STS)内部に、V6は頭頂後頭溝(parieto-occipital sulcus, PO)内部にあり、上視野と下視野が連続した一枚の視野地図を持つ。
非侵襲的な計測法(fMRI)の開発により、視野地図のイメージングによるヒトの領野区分が進んだ。V1、V2、V5/MTのようなマカカ属サルと相同な領野(ホモログ)が同定されているが、V3、V4、V6等の高次領域については諸説ある(後述。V3、V4、V6の項を参照)。ヒトの高次領域では個体差が大きい。ネコやフェレットではV1、V2、V3をそのまま17野、18野、19野と呼ぶことが一般的である[2][3]。ネコやフェレットの高次領域の区分は確立されていない。サルの視覚前野がV1から主な入力を受けるのに対して、ネコやフェレットでは、外側膝状体から17野、18野、19野に並行な投射が存在する[4]。マウスやラットの大脳皮質にもV1より高次の視覚領域が複数存在することが知られているが、個別の領野として確立されるには至っていない[5][6][7][8][9][10][11]。
階層的なネットワークと視覚情報の中間処理
視覚前野の機能的な領野は階層的な結合関係を持ち、V1と高次視覚野(側頭葉、後頭頂葉)の間で、視覚情報の中間処理を行う。領野間のフィードフォワード投射に着目すると視覚情報の流れを階層的なネットワークの枠組みで捉えることができる。V1ニューロンは、その受容野内に呈示される局所的な刺激特徴(色(輝度)、線の傾き、両眼視差による奥行、運動方向)とその位置の情報(受容野の位置)を視覚前野に伝える。視覚前野の階層を上がるにつれて受容野のサイズが大きくなり、より広範囲の視野に含まれる刺激要素や刺激特徴の情報を取捨選択して統合する。より複雑な刺激特徴を抽出するとともに、視野情報から知覚情報への変換課程の一端を担う。ただし、領野間の情報表現の変化には重複も大きく、階層を上がる度に領野の性質が変化するというよりは、複雑な刺激特徴に選択的に反応するニューロンの割合が徐々に増えていくという見方の方が正確である。一方、刺激要素の位置情報やレチノトピーの性質は徐々に失われる。またV2やV4ではCOストライプやグロブ(後述。V2、V4の項を参照)ごとに局所的な視野地図の繰り返しが生じている。視覚情報の流れは主に背側視覚路と腹側視覚路とに分かれる[12][13][14][15][16][17](詳細は視覚経路、受容野を参照)。同一視野の情報が複数の領野で分散並列処理されており、外側膝状体やV1と異なり、視覚前野のある領野が局所的に損傷されても視野に欠損(暗点)は生じない。
背側視覚路
外側膝状体の大細胞系(M経路)由来の入力を受け、その性質(色選択性が無い、輝度コントラスト感度が高い、時間分解能が高い、空間分解能が低い)を引き継ぐ[18][19]。色選択性を持たず、ほとんどのニューロンが運動(方向、速度)や両眼視差に選択性を示す。V2(太い縞)、V3、V5/MT、V6を介して後頭頂葉に出力し、運動や空間構造の認識に関与するとされる。領野間は有髄線維により結合され、伝導速度が速く、ミエリン染色で濃く染まる。V1より各領野へ直接投射があり、視覚刺激の呈示開始よりニューロンの反応が生じるまでの時間(潜時)を比較しても領野間の差がほとんどない[20]。V5/MTのニューロンは等距離平面上のドットパターンの運動方向や注視点を基準とする平面の奥行き(絶対視差)に選択性を示す。V3、V6のニューロンは奥行方向の傾きや3次元方向の運動に選択性を示す。視覚前野が投射する後頭頂葉のうち、MST、VIP、7aはオプティカルフロー(ドットパターンの発散、収縮、回転)などの3次元空間での動きの知覚に関与するとされる(運動視を参照)。一方、視覚前野が投射する後頭頂葉のうち、V6A、LIPは空間の立体構造や3次元空間での位置関係を表し、身体座標による視線の移動や物体の把持操作に利用される。その際には、必ずしも刺激が意識されているわけではない[21]。
腹側視覚路
外側膝状体の大細胞系(M経路)と小細胞系(P経路)から同程度の入力を受け、さらに顆粒細胞系(K経路)由来の入力も受けて[22]、多様な刺激特徴に選択性を示す。V2(細い縞、淡い縞)、V4を介して側頭葉に出力し、輪郭線(形状)や面の特性(色、テクスチャ)による物体認識に関与するとされる。色情報は小細胞系を介して主に腹側視覚路に伝えられるが、V4ニューロンの約半数しか色選択性を示さない。高次の領野ほど潜時が長い[20]。傾きの変化(輪郭線の折れ曲がり(V2)、曲線(円弧、非カルテジアン図形(同心円、らせん、双曲線)、フーリエ図形)(V4))や、両眼視差の変化(受容野内外の相対視差(V2、V4)、3次元方向の線や平面の傾き(V3、V4))に選択性を示す。V1が輝度対比や色対比(色覚を参照)に反応するのに対して、特定の色相や彩度(V2、V4)に選択性を示す。平面のテクスチャやパターン(V4)、自然画像に含まれる高次統計量(V2、V4)に選択性を示す。視覚前野が投射する側頭葉のTEO、TEは、複雑な輪郭線の形状、物体表面の3次元形状、手や顔のようなもっと複雑な刺激を表し、物体の認識や表象(意識に上らせること)に関与するとされる[23][24][25][26]。
重層的なネットワークと視覚情報の修飾
視覚前野には階層的なネットワークの枠組みだけでは説明できない情報の流れが存在する。フィードフォワード投射以外にも、領野内の水平結合や領野間のフィードバック投射の寄与が大きく、背側と腹側の視覚路間にも結合が存在する。そのために視覚経路に沿った大まかな視覚情報の流れとともに、ネットワーク内で視覚情報が収束、拡散、周回を繰り返している。視覚前野のニューロンには、受容野外に呈示される視覚情報による修飾作用を強く受けるもの、視覚刺激の全体が表す大局的な“見え”に選択性を示すニューロンがある。また、注意と予測(後述)や真の運動検出(後述)のように非視覚情報による修飾作用を強く受けるものがある。修飾作用をもたらす情報の入力経路をはじめとして、このような重層的なネットワークのメカニズムはまだよく分っていない。
フィードバック投射による修飾
V2ニューロンへの入力の2/3はV1からの投射であるとされ[27]、V1の活動を抑制するとV2ニューロンは反応しなくなる[28][29]。一方、ほぼ同数の投射があるとされるV2からV1へのフィードバックを遮断すると、V1ニューロンの反応の選択性に顕著な変化はないが[30]、受容野外部に作用する周辺抑制が変化する[31]。V2ニューロンはV1以外にもV4、V5/MT、視床枕(pulvinar)から入力を受けている。これらの入力を遮断すると、V2ニューロンで自発発火頻度や反応強度が経時的に増減する[32]。大きな受容野と複雑な刺激特性を持つ高い階層のニューロンからのフィードバック投射が、低い階層のニューロンの反応選択性の形成に果たす役割はよく分かっていない。
非古典的受容野からの修飾
(古典的)受容野外に呈示される視覚刺激が単独でニューロンを反応させることはないが、刺激特徴やそのパラメータ、受容野内外の刺激要素の組み合わせ方や空間配置により選択的な修飾作用を示すことがある。そうした作用を生じる受容野の周辺部分を非古典的受容野という。V1と同様に、V2のニューロンには、受容野よりも大きなサイズの線やドットパターンを呈示すると反応が抑制されるもの(周辺抑制)、受容野の中と外に同時に呈示された線分間の直列性が強いほど反応が増強(促通)するもの(文脈依存性修飾作用、contextual modulation)[33]がある。V4やV5/MTにも受容野よりも大きなサイズの視覚刺激を呈示すると反応が抑制されるニューロンがあり、古典的受容野の中と外での奥行きや運動(向き、速度)の対比を表すとされる[34][35][36](受容野を参照)。
大局的な情報
知覚される視覚刺激の“見え”は、個々の視覚刺激よりも、視覚刺激全体が表す大局的な情報に従うことがある。視覚前野には、受容野内に呈示された個々の視覚刺激の刺激特徴よりも、受容野の内外に広がる視覚刺激全体が表す刺激特徴の配置や組み合わせに対して選択的に反応するニューロンがある。このことは、視覚前野において、視野情報から知覚情報への変換が始まることを示す。
主観的輪郭線(subjective contour) カニッツァの三角形や縞模様の端部では、線や端点の配列から存在しない面や輪郭線を知覚できる。V2にはこうした主観的輪郭線の傾きにも反応するニューロンがある[37][38][39]。
境界線の帰属(border ownership) 図と背景(地)の境界線は常に“図”の輪郭線として知覚されるが、輪郭線のどちら側が図であるかは視覚刺激全体で決まる。V2には、受容野を横切る輪郭線のどちら側が図であるかに選択的に反応するニューロンがある[40][41]。
逆相関ステレオグラム(anti-correlated stereogram) 点が面状に分布するドットパターンから、その面の奥行きを知覚できる。点の輝度コントラストを左右の目で逆にすると、点は見えても対応付けられず、奥行きをもった面を知覚できなくなる[42]。V2、V4にはある奥行きを持った面に選択的に反応するニューロンがあるが、点刺激の輝度コントラストを左右の目で逆にするとこれらのニューロンの反応が減弱する[43]。
色の恒常性、明るさの恒常性 視覚刺激の波長成分は刺激物体の反射特性と照明光により決まるが、モンドリアン図形のように周囲に異なる色の刺激を同時に呈示すると、照明条件によらずに同じ色相や輝度が知覚される。V4には、受容野の中外に異なる色刺激を同時に呈示すると、照明条件によらず色相や輝度に同じ選択性を示すニューロンがある[44]。
窓枠問題(aperture problem) ある方向に動いている線刺激や縞模様を円形の窓を通して見ると、端点の動きが隠されて実際の運動方向が分からなくなる。この時、運動速度の最も低い、線の法線方向への運動が知覚される。一方、長方形の窓を通して動く縞模様を見ると、長辺沿いの端点の動きが運動方向として知覚される(バーバーポール錯視)。V5/MTのニューロンには、受容野外に長方形の枠を呈示すると、枠沿いの端点の運動方向に選択性を示すものがある[45][46]。
格子模様(plaid pattern) 傾きの異なる二つの縞模様を重ねて動かすと、多くの場合は、格子模様が一方向に動いて見える。その運動方向は二つの縞の法線方向のベクトル和の方向になる。しかし、ふたつの縞模様の奥行きを変えたり、縞の重複部分の輝度を調整して半透明の縞模様が重なっているように見せると、二つの縞模様がすれ違ってそれぞれ別方向に動くようにしか見えない。V5/MTのニューロンには、格子模様が動いて見える条件では格子模様の運動方向に選択的に反応し、縞模様がすれ違うように見せる条件ではそれぞれの縞模様の法線方向に選択的に反応するものがある[47][48][49]。
注意や予測(期待)
注意を向けることにより我々の知覚は視覚情報以外の能動的な修飾作用を受ける(空間的注意、選択的注意を参照)。特定の場所、特定の刺激物体、色や形などの特定の刺激属性に注意を向けさせた状態で神経活動を記録すると、注意を向けていない場合とくらべて、同じ視覚刺激に対する反応の増強(ゲイン)、反応潜時の減少、刺激選択性の向上(応答特性)、受容野の縮小や移動(空間特性)などが観察される[50][51]。このような修飾作用は、V5/MT[52][53][54][55]やV4[56][57][58][59]で顕著であり、V1、V2では弱い[60][61]。局所電場電位の周波数成分解析により、V4では注意が向けられると神経細胞活動の同期性が高まることが報告されている[62]。ヒトでも同様の作用が報告されている[63]。
真の運動の検出
我々は、空間内での物体の真の動き(real motion)と、視線や頭部の動きにより網膜上に生じる見かけの動き(self-induced motion)を区別することができる。そのためには視野上に生じる運動情報から眼球や頭部の運動で生じる運動情報を差し引くことが必要である。背側視覚路のニューロンには見かけの動きよりも真の動きに反応するものがあり(V3A、V6)、頭部座標に基づく真の動きの検出に関与するとされる[64][65][66]。眼球や頭部の運動の情報がどのようにもたらされるのか分かっていない。
知覚の神経メカニズム
視覚前野の領野が特定の刺激特性に関与することから、視覚前野にも知覚判断の中枢として機能する領野が存在することが期待された。運動からの構造の知覚(後述)において知覚される運動方向の変化に合わせてV5/MTのニューロンの反応が変化することと、ドットパターンの運動方向の知覚(後述)において以下の条件を満たすことから、V5/MTのニューロンがそうした視覚中枢の一つであるとされる。しかし、V5/MT以外の領野では、ニューロン活動と個体の知覚判断との因果関係を明らかにする試みはあまり成功していない。
一群のニューロンが特定の視知覚の神経メカニズム(神経相関、neural correlates)であることの根拠として、サルなどの動物を強制選択課題で訓練し、課題遂行中に電気活動を記録して、①ニューロンの反応選択性が知覚判断に必要な情報を十分に表すこと、②試行ごとに動物の知覚判断とニューロンの反応強度の間に相関関係が存在すること、③ある領野を局所的に破壊、麻痺、電気刺激することにより動物の知覚判断を操作できること、④曖昧な視覚刺激に対する試行ごとの知覚判断の変動がニューロンの反応強度の変動と相関すること、⑤知覚判断の表示方法(動作)と無関係であること、などを示す必要がある[67][68][69]。V5/MTでは①領野内の大多数のニューロンが運動方向や両眼視差に選択性を示し、領野として特定の機能に特化していた、②運動方向や奥行に対する選択性が等しいニューロンがコラム状の狭い領域に集中しており、それらの操作が容易であった、③結果的に知覚判断が比較的小数のニューロンの活動に依存していたことが、因果関係を検証する際の利点となったと考えられる。
運動からの構造の知覚(structure from motion)[70] 垂直に立てた透明な円筒の表面にドットパターンを貼り付ける。この円筒を回転させた時に生じる各点の左右の動きを平面なスクリーンに呈示すると、回転する円筒が知覚される。両眼視差の情報がないので、画像からは円筒の前面の点が左右どちら方向に動くかは分からず、知覚される円筒の回転方向は不定期に変化する。知覚される回転方向の変化に合わせて反応強度が変化するニューロンがV5/MTで見つかった。
ドットパターンの運動方向や奥行きの知覚[67][68][69] 各点がランダムに動くドットパターンの中で一定の割合の点が同じ方向に運動する時、その割合(コヒーレンス)が高い程、それらの点が示す運動方向が知覚されやすくなる。コヒーレンスが高いほど運動方向を識別する課題の正答率が高くなることから、正答率により運動の見えを評価できる。記録中のV5/MTニューロンの最適な運動方向あるいはその反対方向へ動く点を含むドット刺激を用い、サルに強制選択課題で2方向から選択させたところ、①コヒーレンスの度合いによりニューロンの反応強度が変化した、②ニューロンの反応強度から運動方向の見えを確率的に推測できた、③V5/MTを局所的に破壊、麻痺、電気刺激してサルの正答率を操作できた、④曖昧な刺激(コヒーレンスなし)に対する知覚判断の試行ごとの変動がニューロンの反応の変動と相関していた(choice-probability)、⑤これらの対応や変調が知覚判断の表示法(視線の移動、手によるレバー押し)によらなかった。これらの結果から、比較的少数のV5/MTニューロンの活動が運動方向の知覚判断を左右することが示された。
視覚情報処理のメカニズム
視覚前野における視覚情報処理のプロセスやメカニズムを解明するには、ニューロンや機能的領野の結合関係、視覚刺激とニューロンの反応特性と知覚判断の間の因果関係に加えて、背後にある計算理論の理解する必要がある(Marrの計算論を参照)。V1ニューロンは視覚情報(画像情報)に対してある種の時空間フィルタ(受容野視差エネルギーモデルを参照)として機能すると考えられるが、視覚前野のモデル研究では階層的なネットワークの枠組みでV1、V2からの入力をもとにV2,V4,V5/MTの刺激選択性がどのように形成されるのかがテーマとなる。各領野のニューロンが示す刺激特徴(輪郭線の形状、面の特性、運動パターン)の検出、さらには修飾作用や反応選択性の不変性(位置、サイズ、手がかり刺激など)を説明する神経モデルが提案されている(詳細は計算論的神経科学を参照)。
計算機技術の進歩に伴い、大規模なモデルのフィッティングや学習によるパラメータやネットワークモデルの最適化と統計学的な解析が可能となった。今日のモデル研究においては、ニューロンが示す反応を定量的に説明する研究が盛んである。当初は、特定の刺激要素(線成分、色(輝度)成分、運動成分、空間周波数成分)への反応を組み合わせるタイプのモデル研究が多かった。例えば、輪郭線の表現であれば、傾き、長さ、位置で表される線分(パーツ)を複数用意し、それらの組み合わせて輪郭線を表現すると考える。これを説明するモデルでは、実際の入力の代わりに、パーツ単体に対するニューロンの反応ないしはパーツを表す入力(例えばV1モデルの出力)をもとに輪郭線に対する反応を予測する。このようなモデルでは、個々のニューロンの反応に対してはモデルのパラメータを最適化する[71][72][73][74]。
また、重層的なネットワークの性質として、フィードバック投射や受容外から作用する興奮性/抑制性の修飾作用を取り入れたモデルも提案されている[75][76][77]。その後、ニューラルネットワークのように入力層(例えばV1モデルの2次元配列)の出力を組み合わせるタイプのモデル研究が多くなった。ドットパターン、テクスチャ、自然画像に特定の刺激要素を見いだすことは難しいが、時空間フィルタ(V1モデル)の出力を組み合わせることで、視覚刺激に含まれる空間周波数成分の分布や高次統計量が寄与することが示された(V2,V4)[78][79][80][81][82]。
近年では、不特定多数の自然画像を視覚刺激とするデータ駆動型の解析も増えている。スパース符号化(sparse coding)や深層学習をキーワードにして、ネットワークモデル自体を最適化するタイプの研究が盛んになっている[83][84][85][86][87]。
近年、深層ニューラルネットワークによる視覚情報処理技術が著しく進歩している(詳細は人工知能を参照)。その中間層(隠れ層)のノードがV1ないしV4のような特性を持つことが示されている[88]。脳のメカニズムをそのまま再現することが目的のネットワークモデルではないが、モデルのリバースエンジニアリングが視覚前野のメカニズム研究の手がかりを与えることが期待される[89]。
各領野の解剖学的特徴とその機能
V2野
18野の一部。V1に隣接する帯状の領域。背側部が反対側の下視野を、腹側部が反対側の上視野を表す。V1の主な出力先である。V1から主な入力を受け、V1 へ強いフィードバック投射する。V3、V4、V5/MTへ出力する。V1以外にMT、V4からのフィードバック入力および視床枕(pulvinar)から入力を受ける。
チトクローム酸化酵素(CO)により染色すると、太い縞(thick stripe)、細い縞(thin stripe)、淡い縞(inter stripe、pale stripe)の縞状の領域(COストライプ)に区分され、外側から内側へ淡―太―淡―細と縞領域が繰り返し分布する[90][91][92]。太い縞はV1(4b層)より大細胞系の入力を受け、V3、MTに投射するので、背側視覚路に属するとされている。太い縞のニューロンは運動方向、速度、両眼視差に選択性を示す。細い縞はV1(ブロブ領域)より入力を受けV4に投射するので、腹側視覚路に属するとされている。細い縞のニューロンは色相に選択性を示す。淡い縞はV1(2/3層のブロブ間隙領域)より小細胞系の入力を受け、V4に投射するので、腹側視覚路に属するとされている。淡い縞のニューロンは線の傾きに選択に反応し、エンドストップ抑制により端点を表す。
Livingston以降、V2が3つの視覚経路(太い縞、細い縞、淡い縞)に分かれており、それぞれが運動、色、形の情報処理を分担するとされてきた[93]。しかし、Sincichらによれば①細い縞はV1の2/3層のブロブ領域以外に4a, 4b, 5/6層からも入力を受け、②太い縞と淡い縞は2/3層のブロブ間隙領域と4a,4b,5/6層から入力を受けて、線の傾きに選択性を示し、③太い縞と淡い縞の違いは出力先(V4、V5/MT)の違いであるという。従って、各縞が受け取る情報の差はそれほど明瞭でなく、V1のブロブ領域とブロブ間隙領域に発する2つの経路に大別されるという[94]。また、太い縞の外側に隣接する淡い縞にはV1(4b層)からの入力があり太い縞と似た反応を示し、太い縞の内側に隣接する淡い縞と区別されることが示された[95]。
V2のニューロンはV1のニューロンよりも概して低い空間周波数成分によく反応する。大局的な情報(主観的輪郭線の傾き、輪郭線を挟んだ図と地の向き、逆相関ステレオグラム)、ドットパターンの面の奥行き段差が示す境界線の傾き[96]、受容野を横切る輪郭線の折れ曲がり[97][98]、傾きや周波数成分の異なる縞模様の組み合わせ[99]、自然画像に含まれる高次の統計量成分[80][81]などに選択性を示すニューロンがある。
V3野
18野の一部。V2に隣接する帯状の領域である。腹側と背側は異なる2つの領域であるとする説もある。主に旧世界ザルを対象とした研究では背側部(V3d)と腹側部(V3v)を合わせて一つのV3であるとされる[100][101]。新世界ザル(ヨザルなど)では背側部(DM)の一部がV3に相当し[88]、腹側部はVP野(腹側後部領域、ventral posterior area)と呼ばれる別の領域であるとされる[102][103][104][18]。マーモセットでは腹側部と背側をあわせてVLPと呼ぶ。
腹側部はV2(細い縞、淡い縞)から入力を受け、側頭葉(V4、VTF、VOF)に投射するので、腹側視覚路に属するとされている。反対側の上視野を表す。ニューロンは色選択性を示す。背側部はV2(太い縞)とV1(4b層)から入力を受け、V3a、V4、V5/MT、V6と後頭頂葉(DP,VIP,LIP)に出力するので、背側皮質視覚路に属するとされている。反対側の下視野を表す。ミエリン染色で濃く染まり、ニューロンは輝度や奥行きに選択性を示すが、色選択性を示さない。広域的な動きや奥行き方向の傾き、テクスチャの充填(欠損部の補完))[105]に関わる。
V2とV4の間の領域を第3次視覚皮質複合体と総称する。ヒトでよく発達しており、サルとの違いが顕著な領域である。V3AはV3d前方に隣接し、上視野と下視野をあわせた視野地図を持つ領野である。V1、V2、V3dより入力を受け、MT、MST、LIPへ出力する。サルのV3AはV3dよりも速度や奥行きに選択性を示すニューロンが少なく、ドットパターンよりも線刺激に強く反応する。注意の効果が顕著に見られる[106]。視線の向きによらずに、頭部の向きを基準とする位置に選択性を示すものがある[64]。一方、ヒトのV3AはV3dよりもドットパターンで表される運動刺激によく反応し、経頭蓋電気刺激(TMS)を与えると速度の知覚が障害される[107]。V3Bはヒトに存在する領域で[108][109]、両眼視差による奥行き表現と運動視差による奥行き表現が統合されている[110]。しかし、サルではこの統合はV5/MTに生じる。V3A,V3Bとも主に周辺視野を表す[111]。
V4野
19野の一部。V3に隣接する領域。背側部(V4d)と腹側部(V4v)を合わせて一つのV4とする。背側部は上視野の垂直子午線に近い部分を表す。腹側部は上視野の水平子午線に近い部分を含む残りの視野を表す。新世界ザルの背外側野(DL)、マーモセットのVLAに相当する。V2(細い縞、淡い縞)、V3、V3Aから強い入力を受け、側頭葉(TEO、TE)、後頭頂葉(MT、MST、FST、V4t、DP、VIP、LIP、PIP)、前頭葉(FEF)へ出力する。V1、V2、V3にフィードバック投射を返す。中心視領域はV1から直接投射を受け[112]、側頭葉(TEO、TE)と強い結合を持つ。周辺視領域はV3、V5/MTから強い入力を受け、後頭頂葉からも広く入力を受ける。
多くの領野と結合しており、多様な機能を持つ[113][114]。1970年代には、色に選択的なニューロンが多く、その一部が色恒常性を示すことから、V4が色表現の中枢であるとする説が提案された[100][115]。しかし、1980年代になると輪郭線の傾きに選択性を示すニューロンも多数あることが明らかにされた[116][34][117]。近年、色と形のサブ領域(グロブ)に分かれることが示されている[118][119]。他にも、曲線(円弧、非カルテジアン図形(同心円、らせん、双曲線))[120][121][122]、曲線の曲率と傾きの組み合わせ[123][71]、縞模様の空間周波数成分と傾きの組み合わせ、3次元方向の線の傾き[124]、受容野内外の相対的な奥行き(relative disparity)[125]、ドットパターンの印影方向[126]、自然画像に含まれる高次の統計量成分[82]などに選択性を示すニューロンがある。さらに、大局的な選択性(色恒常性、逆相関ステレオグラム)を示すもの、注意により強い修飾作用を受けるもの、複雑な輪郭線の形状に選択性を示すもの[25][26]、視覚刺激の位置、大きさ、刺激手がかりの変化に対して反応選択性の不変性を示すもの[127][128][129][71]などのニューロンがある。
サルのV4を破壊すると、①大きさの変化、遮蔽、色恒常性、主観的輪郭線に対応できなくなる、②混在している複数の刺激要素を区別することができなくなる、③同一物体の持つ奥行き,明暗,色,位置などの情報を同一物体のものとして関連付けることができなくなる[130][131][132][133]などの影響が生じる。
fMRIによるヒトV4の研究では背側部に相当する領域が同定されず、以下の諸説がある[111][134]。①V4には下視野をあらわす領域(背側部)が存在しない。V3dに隣接する領域(LO1,LO2)はそれぞれ全視野を表す別の領域である。②背側部は存在しない。腹側のV8がV4の一部で下視野を表し、合わせて全視野を表す一つの領域である。③背側部は存在する。fMRIの空間分解能を上げると、腹側部よりも面積が小さく、主に下視野の中心視野部分を表す領域が同定されるので、これを背側部とする。下視野の周辺視野を含む残りの視野は腹側部で表される。ヒトのV8については、V8が損傷を受けると色覚だけが失われることからV4の一部とする説と、サルのTEOに相当する別の領域とする説がある[134][135][136][137]。
V5/MT野
19野の一部。視覚刺激の運動方向に選択性をもつニューロンが多数ある領域(V5)とミエリン染色で濃く染まる領域(MT、middle temporal area)として別々に同定されたが、後に同じ領域であることが明かにされた[138][139]。チトクローム酸化酵素[140]やCat301抗体[141]で濃く染まる。ヒトでは、隣接する領域(MST等)と合わせて、MT complex、hMT、MT+、V5/MTと呼ばれることが多い[142][143]。上視野と下視野をあわせた視野地図を持つ。背側視覚路に属し、主にV1(4b層)より、他にV2(太い縞)、V1(6層)、V3背側部、V4、V6から入力を受ける[1][144]。周辺視の領域は脳梁膨大後部皮質からも入力を受ける[145]。主に隣接するMST、FST、V4tへ、他に前頭眼野(FEF)、頭頂間溝(LIP、VIP)、上丘(SC)へ出力する。また、外側膝状体、視床枕から直接入力を受ける[146](盲視を参照)。
大部分(70-85%)のニューロンが視覚刺激の運動方向、速度、両眼視差に選択性を示し[47][139][147][148]、運動方向と両眼視差の機能的コラム(V1を参照)が存在する[149][150]。V5/MTのニューロンには、注視面からの絶対視差(absolute disparity)に選択性を示して奥行きの異なる面を区別するもの、運動視差(自己運動に伴う、奥行きの違いにより生じる見かけの運動速度や運動方向の変化)に選択性を示すもの、運動方向の違いだけで示される境界線に選択性を示すもの、3次元方向への運動に選択性を示すものがある[151][152]。両眼視差による奥行き表現と運動視差による奥行き表現は、サルではV5/MTで、ヒトではV3Bで統合される[110]。注意により強い修飾を受ける。
サルのV5/MTが運動知覚の中枢として機能することが示されている(知覚の神経メカニズムの項を参照)。ヒトのV5/MTが損傷されると、刺激刺激の運動に追従して生じる眼球運動が障害され、運動を知覚できずに世界が静的な"フレーム"の連続に感じられる[153][154][155](詳細は視覚失認、運動盲を参照)。V5/MTに経頭蓋磁気刺激を与えると視覚刺激の運動の知覚が阻害される[156]。
V6野
19野の一部。頭頂後頭溝(parieto-occipital sulcus)前壁に位置し、V2、V3に隣接する、上視野と下視野をあわせた視野地図を持つ領域。PO野[157][158][159]とも呼ばれる。後に頭頂後頭溝前壁の腹側部分の視覚領域(V6野)と背側部分の視覚―運動領域(V6A野)に区別された[160][161][162]。ミエリン染色で濃く染まる[163]]。新世界ザルでは背内側野(DM)の一部が相当する。当初はヒトや旧世界ザル(マカカ属サル)には存在しないとされた。ヒトのV6は頭頂後頭溝の最背側部に位置する[164]。後頭葉(V1、V2、V3、V3A、V5/MT)と後頭頂葉(V6A、VIP)とに双方向の結合を持つ[165]。V1(Ⅳb)より大細胞系の入力を直接受けている。背側皮質視覚路に属するとされる。他の領野と異なり、周辺視野に移っても領野内の占有面積の割合は変わらない。V6のニューロンにはエンドストップ抑制が弱く低空間周波数成分に反応するもの、大きなエッジの動きに方向選択的に反応するものがある。ヒトのV6は大きなドットパターンの3次元方向の運動やフリッカー刺激に反応する[164][166][167]。ヒトのV6を含む部位が損傷されると、運動方向の区別あるいは運動自体の検出が阻害される[168]。ヒト、サルのV6には、空間中の物体の真の動きには反応するが、目や頭部の動きにより網膜上に生じる見かけの動きに反応しないニューロンがある[64][65][66]。
関連項目
参考文献
- ↑ 1.0 1.1
Felleman, D.J., & Van Essen, D.C. (1991).
Distributed hierarchical processing in the primate cerebral cortex. Cerebral cortex (New York, N.Y. : 1991), 1(1), 1-47. [PubMed:1822724] [WorldCat] [DOI] - ↑
Payne, B.R. (1993).
Evidence for visual cortical area homologs in cat and macaque monkey. Cerebral cortex (New York, N.Y. : 1991), 3(1), 1-25. [PubMed:8439738] [WorldCat] [DOI] - ↑
Manger, P.R., Kiper, D., Masiello, I., Murillo, L., Tettoni, L., Hunyadi, Z., & Innocenti, G.M. (2002).
The representation of the visual field in three extrastriate areas of the ferret (Mustela putorius) and the relationship of retinotopy and field boundaries to callosal connectivity. Cerebral cortex (New York, N.Y. : 1991), 12(4), 423-37. [PubMed:11884357] [WorldCat] [DOI] - ↑
Stone, J., Dreher, B., & Leventhal, A. (1979).
Hierarchical and parallel mechanisms in the organization of visual cortex. Brain research, 180(3), 345-94. [PubMed:231475] [WorldCat] [DOI] - ↑
Caviness, V.S. (1975).
Architectonic map of neocortex of the normal mouse. The Journal of comparative neurology, 164(2), 247-63. [PubMed:1184785] [WorldCat] [DOI] - ↑
Schnagl, R.D., Holmes, I.H., & Mackay-Scollay, E.M. (1978).
Coronavirus-like particles in Aboriginals and non-Aboriginals in Western Australia. The Medical journal of Australia, 1(6), 307-9. [PubMed:661689] [WorldCat] - ↑
Wagor, E., Mangini, N.J., & Pearlman, A.L. (1980).
Retinotopic organization of striate and extrastriate visual cortex in the mouse. The Journal of comparative neurology, 193(1), 187-202. [PubMed:6776164] [WorldCat] [DOI] - ↑
Coogan, T.A., & Burkhalter, A. (1990).
Conserved patterns of cortico-cortical connections define areal hierarchy in rat visual cortex. Experimental brain research, 80(1), 49-53. [PubMed:2358036] [WorldCat] [DOI] - ↑
Coogan, T.A., & Burkhalter, A. (1993).
Hierarchical organization of areas in rat visual cortex. The Journal of neuroscience : the official journal of the Society for Neuroscience, 13(9), 3749-72. [PubMed:7690066] [WorldCat] - ↑
Montero, V.M. (1993).
Retinotopy of cortical connections between the striate cortex and extrastriate visual areas in the rat. Experimental brain research, 94(1), 1-15. [PubMed:8335065] [WorldCat] [DOI] - ↑
Wang, Q., & Burkhalter, A. (2007).
Area map of mouse visual cortex. The Journal of comparative neurology, 502(3), 339-57. [PubMed:17366604] [WorldCat] [DOI] - ↑ L G Ungerleider, M Mishkin
Two cortical visual systems.
Analysis of Visual Behavior (D J Ingle, M A Goodale, R J W Masfield, eds.), MIT Press, Cambridge, MA, 1982. - ↑
DeYoe, E.A., & Van Essen, D.C. (1988).
Concurrent processing streams in monkey visual cortex. Trends in neurosciences, 11(5), 219-26. [PubMed:2471327] [WorldCat] [DOI] - ↑
Gattass, R., Rosa, M.G., Sousa, A.P., Piñon, M.C., Fiorani Júnior, M., & Neuenschwander, S. (1990).
Cortical streams of visual information processing in primates. Brazilian journal of medical and biological research = Revista brasileira de pesquisas medicas e biologicas, 23(5), 375-93. [PubMed:1965642] [WorldCat] - ↑
Baizer, J.S., Ungerleider, L.G., & Desimone, R. (1991).
Organization of visual inputs to the inferior temporal and posterior parietal cortex in macaques. The Journal of neuroscience : the official journal of the Society for Neuroscience, 11(1), 168-90. [PubMed:1702462] [WorldCat] - ↑
Van Essen, D.C., Anderson, C.H., & Felleman, D.J. (1992).
Information processing in the primate visual system: an integrated systems perspective. Science (New York, N.Y.), 255(5043), 419-23. [PubMed:1734518] [WorldCat] [DOI] - ↑
Ungerleider, L.G., & Haxby, J.V. (1994).
'What' and 'where' in the human brain. Current opinion in neurobiology, 4(2), 157-65. [PubMed:8038571] [WorldCat] [DOI] - ↑ 18.0 18.1
Burkhalter, A., & Van Essen, D.C. (1986).
Processing of color, form and disparity information in visual areas VP and V2 of ventral extrastriate cortex in the macaque monkey. The Journal of neuroscience : the official journal of the Society for Neuroscience, 6(8), 2327-51. [PubMed:3746412] [WorldCat] - ↑
Levitt, J.B., Kiper, D.C., & Movshon, J.A. (1994).
Receptive fields and functional architecture of macaque V2. Journal of neurophysiology, 71(6), 2517-42. [PubMed:7931532] [WorldCat] [DOI] - ↑ 20.0 20.1
Schmolesky, M.T., Wang, Y., Hanes, D.P., Thompson, K.G., Leutgeb, S., Schall, J.D., & Leventhal, A.G. (1998).
Signal timing across the macaque visual system. Journal of neurophysiology, 79(6), 3272-8. [PubMed:9636126] [WorldCat] [DOI] - ↑
Goodale, M.A., & Milner, A.D. (1992).
Separate visual pathways for perception and action. Trends in neurosciences, 15(1), 20-5. [PubMed:1374953] [WorldCat] [DOI] - ↑
Maunsell, J.H. (1992).
Functional visual streams. Current opinion in neurobiology, 2(4), 506-10. [PubMed:1525550] [WorldCat] [DOI] - ↑
Desimone, R., Albright, T.D., Gross, C.G., & Bruce, C. (1984).
Stimulus-selective properties of inferior temporal neurons in the macaque. The Journal of neuroscience : the official journal of the Society for Neuroscience, 4(8), 2051-62. [PubMed:6470767] [WorldCat] - ↑
Fujita, I., Tanaka, K., Ito, M., & Cheng, K. (1992).
Columns for visual features of objects in monkey inferotemporal cortex. Nature, 360(6402), 343-6. [PubMed:1448150] [WorldCat] [DOI] - ↑ 25.0 25.1
Kobatake, E., & Tanaka, K. (1994).
Neuronal selectivities to complex object features in the ventral visual pathway of the macaque cerebral cortex. Journal of neurophysiology, 71(3), 856-67. [PubMed:8201425] [WorldCat] [DOI] - ↑ 26.0 26.1
Hegdé, J., & Van Essen, D.C. (2007).
A comparative study of shape representation in macaque visual areas v2 and v4. Cerebral cortex (New York, N.Y. : 1991), 17(5), 1100-16. [PubMed:16785255] [WorldCat] [DOI] - ↑
Sincich, L.C., & Horton, J.C. (2003).
Independent projection streams from macaque striate cortex to the second visual area and middle temporal area. The Journal of neuroscience : the official journal of the Society for Neuroscience, 23(13), 5684-92. [PubMed:12843271] [PMC] [WorldCat] - ↑
Schiller, P.H., & Malpeli, J.G. (1977).
The effect of striate cortex cooling on area 18 cells in the monkey. Brain research, 126(2), 366-9. [PubMed:405082] [WorldCat] [DOI] - ↑
Girard, P., & Bullier, J. (1989).
Visual activity in area V2 during reversible inactivation of area 17 in the macaque monkey. Journal of neurophysiology, 62(6), 1287-302. [PubMed:2600626] [WorldCat] [DOI] - ↑
Sandell, J.H., & Schiller, P.H. (1982).
Effect of cooling area 18 on striate cortex cells in the squirrel monkey. Journal of neurophysiology, 48(1), 38-48. [PubMed:6288886] [WorldCat] [DOI] - ↑
Nassi, J.J., Lomber, S.G., & Born, R.T. (2013).
Corticocortical feedback contributes to surround suppression in V1 of the alert primate. The Journal of neuroscience : the official journal of the Society for Neuroscience, 33(19), 8504-17. [PubMed:23658187] [PMC] [WorldCat] [DOI] - ↑
Correia, A.R.A., Amorim, A.K.J., Soares, J.G.M., Lima, B., Fiorani, M., & Gattass, R. (2021).
The role of feedback projections in feature tuning and neuronal excitability in the early primate visual system. Brain structure & function. [PubMed:34089102] [WorldCat] [DOI] - ↑
Bakin, J.S., Nakayama, K., & Gilbert, C.D. (2000).
Visual responses in monkey areas V1 and V2 to three-dimensional surface configurations. The Journal of neuroscience : the official journal of the Society for Neuroscience, 20(21), 8188-98. [PubMed:11050142] [PMC] [WorldCat] - ↑ 34.0 34.1
Schein, S.J., & Desimone, R. (1990).
Spectral properties of V4 neurons in the macaque. The Journal of neuroscience : the official journal of the Society for Neuroscience, 10(10), 3369-89. [PubMed:2213146] [WorldCat] - ↑
Xiao, D.K., Raiguel, S., Marcar, V., Koenderink, J., & Orban, G.A. (1995).
Spatial heterogeneity of inhibitory surrounds in the middle temporal visual area. Proceedings of the National Academy of Sciences of the United States of America, 92(24), 11303-6. [PubMed:7479984] [PMC] [WorldCat] [DOI] - ↑
Craft, E., Schütze, H., Niebur, E., & von der Heydt, R. (2007).
A neural model of figure-ground organization. Journal of neurophysiology, 97(6), 4310-26. [PubMed:17442769] [WorldCat] [DOI] - ↑
von der Heydt, R., Peterhans, E., & Baumgartner, G. (1984).
Illusory contours and cortical neuron responses. Science (New York, N.Y.), 224(4654), 1260-2. [PubMed:6539501] [WorldCat] [DOI] - ↑
von der Heydt, R., & Peterhans, E. (1989).
Mechanisms of contour perception in monkey visual cortex. I. Lines of pattern discontinuity. The Journal of neuroscience : the official journal of the Society for Neuroscience, 9(5), 1731-48. [PubMed:2723747] [WorldCat] - ↑
Peterhans, E., & von der Heydt, R. (1989).
Mechanisms of contour perception in monkey visual cortex. II. Contours bridging gaps. The Journal of neuroscience : the official journal of the Society for Neuroscience, 9(5), 1749-63. [PubMed:2723748] [WorldCat] - ↑
Zhou, H., Friedman, H.S., & von der Heydt, R. (2000).
Coding of border ownership in monkey visual cortex. The Journal of neuroscience : the official journal of the Society for Neuroscience, 20(17), 6594-611. [PubMed:10964965] [PMC] [WorldCat] - ↑
Qiu, F.T., & von der Heydt, R. (2005).
Figure and ground in the visual cortex: v2 combines stereoscopic cues with gestalt rules. Neuron, 47(1), 155-66. [PubMed:15996555] [PMC] [WorldCat] [DOI] - ↑
Tanabe, S., Yasuoka, S., & Fujita, I. (2008).
Disparity-energy signals in perceived stereoscopic depth. Journal of vision, 8(3), 22.1-10. [PubMed:18484828] [WorldCat] [DOI] - ↑
Tanabe, S., Umeda, K., & Fujita, I. (2004).
Rejection of false matches for binocular correspondence in macaque visual cortical area V4. The Journal of neuroscience : the official journal of the Society for Neuroscience, 24(37), 8170-80. [PubMed:15371518] [PMC] [WorldCat] [DOI] - ↑
Zeki, S. (1983).
The distribution of wavelength and orientation selective cells in different areas of monkey visual cortex. Proceedings of the Royal Society of London. Series B, Biological sciences, 217(1209), 449-70. [PubMed:6134287] [WorldCat] [DOI] - ↑ J A Movshon, E H Adelson, M S Gizzi, W T Newsome
The analysis of moving visual patterns.
Study Group on Pattern Recognition Mechanisms (C Chagas, R Gattas, C Gross, eds. Vatican City: Pontifica Academia Scientiarum, pp.117-151,1985. - ↑
Pack, C.C., Gartland, A.J., & Born, R.T. (2004).
Integration of Contour and Terminator Signals in Visual Area MT of Alert Macaque. The Journal of neuroscience : the official journal of the Society for Neuroscience, 24(13), 3268-80. [PubMed:15056706] [PMC] [WorldCat] [DOI] - ↑ 47.0 47.1
Albright, T.D. (1984).
Direction and orientation selectivity of neurons in visual area MT of the macaque. Journal of neurophysiology, 52(6), 1106-30. [PubMed:6520628] [WorldCat] [DOI] - ↑
Rodman, H.R., & Albright, T.D. (1987).
Coding of visual stimulus velocity in area MT of the macaque. Vision research, 27(12), 2035-48. [PubMed:3447355] [WorldCat] [DOI] - ↑
Stoner, G.R., & Albright, T.D. (1992).
Neural correlates of perceptual motion coherence. Nature, 358(6385), 412-4. [PubMed:1641024] [WorldCat] [DOI] - ↑
Desimone, R., & Duncan, J. (1995).
Neural mechanisms of selective visual attention. Annual review of neuroscience, 18, 193-222. [PubMed:7605061] [WorldCat] [DOI] - ↑
Maunsell, J.H., & Cook, E.P. (2002).
The role of attention in visual processing. Philosophical transactions of the Royal Society of London. Series B, Biological sciences, 357(1424), 1063-72. [PubMed:12217174] [PMC] [WorldCat] [DOI] - ↑
Treue, S., & Maunsell, J.H. (1996).
Attentional modulation of visual motion processing in cortical areas MT and MST. Nature, 382(6591), 539-41. [PubMed:8700227] [WorldCat] [DOI] - ↑
Treue, S., & Martínez Trujillo, J.C. (1999).
Feature-based attention influences motion processing gain in macaque visual cortex. Nature, 399(6736), 575-9. [PubMed:10376597] [WorldCat] [DOI] - ↑
Treue, S., & Maunsell, J.H. (1999).
Effects of attention on the processing of motion in macaque middle temporal and medial superior temporal visual cortical areas. The Journal of neuroscience : the official journal of the Society for Neuroscience, 19(17), 7591-602. [PubMed:10460265] [PMC] [WorldCat] - ↑
Seidemann, E., & Newsome, W.T. (1999).
Effect of spatial attention on the responses of area MT neurons. Journal of neurophysiology, 81(4), 1783-94. [PubMed:10200212] [WorldCat] [DOI] - ↑
Moran, J., & Desimone, R. (1985).
Selective attention gates visual processing in the extrastriate cortex. Science (New York, N.Y.), 229(4715), 782-4. [PubMed:4023713] [WorldCat] [DOI] - ↑
Connor, C.E., Preddie, D.C., Gallant, J.L., & Van Essen, D.C. (1997).
Spatial attention effects in macaque area V4. The Journal of neuroscience : the official journal of the Society for Neuroscience, 17(9), 3201-14. [PubMed:9096154] [WorldCat] - ↑
McAdams, C.J., & Maunsell, J.H. (1999).
Effects of attention on orientation-tuning functions of single neurons in macaque cortical area V4. The Journal of neuroscience : the official journal of the Society for Neuroscience, 19(1), 431-41. [PubMed:9870971] [PMC] [WorldCat] - ↑
Reynolds, J.H., Pasternak, T., & Desimone, R. (2000).
Attention increases sensitivity of V4 neurons. Neuron, 26(3), 703-14. [PubMed:10896165] [WorldCat] [DOI] - ↑
Luck, S.J., Chelazzi, L., Hillyard, S.A., & Desimone, R. (1997).
Neural mechanisms of spatial selective attention in areas V1, V2, and V4 of macaque visual cortex. Journal of neurophysiology, 77(1), 24-42. [PubMed:9120566] [WorldCat] [DOI] - ↑
Reynolds, J.H., Chelazzi, L., & Desimone, R. (1999).
Competitive mechanisms subserve attention in macaque areas V2 and V4. The Journal of neuroscience : the official journal of the Society for Neuroscience, 19(5), 1736-53. [PubMed:10024360] [PMC] [WorldCat] - ↑
Fries, P., Reynolds, J.H., Rorie, A.E., & Desimone, R. (2001).
Modulation of oscillatory neuronal synchronization by selective visual attention. Science (New York, N.Y.), 291(5508), 1560-3. [PubMed:11222864] [WorldCat] [DOI] - ↑
Kastner, S., De Weerd, P., Desimone, R., & Ungerleider, L.G. (1998).
Mechanisms of directed attention in the human extrastriate cortex as revealed by functional MRI. Science (New York, N.Y.), 282(5386), 108-11. [PubMed:9756472] [WorldCat] [DOI] - ↑ 64.0 64.1 64.2
Galletti, C., Battaglini, P.P., & Fattori, P. (1990).
'Real-motion' cells in area V3A of macaque visual cortex. Experimental brain research, 82(1), 67-76. [PubMed:2257915] [WorldCat] [DOI] - ↑ 65.0 65.1
Galletti, C., Battaglini, P.P., & Fattori, P. (1995).
Eye position influence on the parieto-occipital area PO (V6) of the macaque monkey. The European journal of neuroscience, 7(12), 2486-501. [PubMed:8845954] [WorldCat] [DOI] - ↑ 66.0 66.1
Fischer, E., Bülthoff, H.H., Logothetis, N.K., & Bartels, A. (2012).
Human areas V3A and V6 compensate for self-induced planar visual motion. Neuron, 73(6), 1228-40. [PubMed:22445349] [WorldCat] [DOI] - ↑ 67.0 67.1
Britten, K.H., Shadlen, M.N., Newsome, W.T., & Movshon, J.A. (1992).
The analysis of visual motion: a comparison of neuronal and psychophysical performance. The Journal of neuroscience : the official journal of the Society for Neuroscience, 12(12), 4745-65. [PubMed:1464765] [WorldCat] - ↑ 68.0 68.1
Salzman, C.D., Murasugi, C.M., Britten, K.H., & Newsome, W.T. (1992).
Microstimulation in visual area MT: effects on direction discrimination performance. The Journal of neuroscience : the official journal of the Society for Neuroscience, 12(6), 2331-55. [PubMed:1607944] [WorldCat] - ↑ 69.0 69.1
Newsome, W.T., & Paré, E.B. (1988).
A selective impairment of motion perception following lesions of the middle temporal visual area (MT). The Journal of neuroscience : the official journal of the Society for Neuroscience, 8(6), 2201-11. [PubMed:3385495] [WorldCat] - ↑
Bradley, D.C., Chang, G.C., & Andersen, R.A. (1998).
Encoding of three-dimensional structure-from-motion by primate area MT neurons. Nature, 392(6677), 714-7. [PubMed:9565031] [WorldCat] [DOI] - ↑ 71.0 71.1 71.2
Pasupathy, A., & Connor, C.E. (2001).
Shape representation in area V4: position-specific tuning for boundary conformation. Journal of neurophysiology, 86(5), 2505-19. [PubMed:11698538] [WorldCat] [DOI] - ↑
Pasupathy, A., & Connor, C.E. (2002).
Population coding of shape in area V4. Nature neuroscience, 5(12), 1332-8. [PubMed:12426571] [WorldCat] [DOI] - ↑
Heeger, D.J., Simoncelli, E.P., & Movshon, J.A. (1996).
Computational models of cortical visual processing. Proceedings of the National Academy of Sciences of the United States of America, 93(2), 623-7. [PubMed:8570605] [PMC] [WorldCat] [DOI] - ↑
Rust, N.C., Mante, V., Simoncelli, E.P., & Movshon, J.A. (2006).
How MT cells analyze the motion of visual patterns. Nature neuroscience, 9(11), 1421-31. [PubMed:17041595] [WorldCat] [DOI] - ↑
Ito, M., & Goda, N. (2011).
Mechanisms underlying the representation of angles embedded within contour stimuli in area V2 of macaque monkeys. The European journal of neuroscience, 33(1), 130-42. [PubMed:21091803] [WorldCat] [DOI] - ↑
Sakai, K., & Nishimura, H. (2006).
Surrounding suppression and facilitation in the determination of border ownership. Journal of cognitive neuroscience, 18(4), 562-79. [PubMed:16768360] [WorldCat] [DOI] - ↑
Peterhans, E., & von der Heydt, R. (1993).
Functional organization of area V2 in the alert macaque. The European journal of neuroscience, 5(5), 509-24. [PubMed:8261126] [WorldCat] [DOI] - ↑
David, S.V., Hayden, B.Y., & Gallant, J.L. (2006).
Spectral receptive field properties explain shape selectivity in area V4. Journal of neurophysiology, 96(6), 3492-505. [PubMed:16987926] [WorldCat] [DOI] - ↑
Naselaris, T., Prenger, R.J., Kay, K.N., Oliver, M., & Gallant, J.L. (2009).
Bayesian reconstruction of natural images from human brain activity. Neuron, 63(6), 902-15. [PubMed:19778517] [PMC] [WorldCat] [DOI] - ↑ 80.0 80.1
Freeman, J., & Simoncelli, E.P. (2011).
Metamers of the ventral stream. Nature neuroscience, 14(9), 1195-201. [PubMed:21841776] [PMC] [WorldCat] [DOI] - ↑ 81.0 81.1
Freeman, J., Ziemba, C.M., Heeger, D.J., Simoncelli, E.P., & Movshon, J.A. (2013).
A functional and perceptual signature of the second visual area in primates. Nature neuroscience, 16(7), 974-81. [PubMed:23685719] [PMC] [WorldCat] [DOI] - ↑ 82.0 82.1
Okazawa, G., Tajima, S., & Komatsu, H. (2015).
Image statistics underlying natural texture selectivity of neurons in macaque V4. Proceedings of the National Academy of Sciences of the United States of America, 112(4), E351-60. [PubMed:25535362] [PMC] [WorldCat] [DOI] - ↑
Cadieu, C., Kouh, M., Pasupathy, A., Connor, C.E., Riesenhuber, M., & Poggio, T. (2007).
A model of V4 shape selectivity and invariance. Journal of neurophysiology, 98(3), 1733-50. [PubMed:17596412] [WorldCat] [DOI] - ↑
Hosoya, H., & Hyvärinen, A. (2015).
A Hierarchical Statistical Model of Natural Images Explains Tuning Properties in V2. The Journal of neuroscience : the official journal of the Society for Neuroscience, 35(29), 10412-28. [PubMed:26203137] [PMC] [WorldCat] [DOI] - ↑
Hatori, Y., Mashita, T., & Sakai, K. (2016).
Sparse coding generates curvature selectivity in V4 neurons. Journal of the Optical Society of America. A, Optics, image science, and vision, 33(4), 527-37. [PubMed:27140760] [WorldCat] [DOI] - ↑
Tao, X., Zhang, B., Smith, E.L., Nishimoto, S., Ohzawa, I., & Chino, Y.M. (2012).
Local sensitivity to stimulus orientation and spatial frequency within the receptive fields of neurons in visual area 2 of macaque monkeys. Journal of neurophysiology, 107(4), 1094-110. [PubMed:22114163] [PMC] [WorldCat] [DOI] - ↑ H Lee, C Ekanadham, A Y Ng
Sparse deep belief net model for visual area V2.
Advances Neural Information Processing Systems, Vol.20 (J C Platt, D Koller, Y Singer, S T Roweis, eds. ,pp873-880,2008. - ↑
Pospisil, D.A., Pasupathy, A., & Bair, W. (2018).
'Artiphysiology' reveals V4-like shape tuning in a deep network trained for image classification. eLife, 7. [PubMed:30570484] [PMC] [WorldCat] [DOI] - ↑
Zhuang, C., Wang, Y., Yamins, D., & Hu, X. (2017).
Deep Learning Predicts Correlation between a Functional Signature of Higher Visual Areas and Sparse Firing of Neurons. Frontiers in computational neuroscience, 11, 100. [PubMed:29163117] [PMC] [WorldCat] [DOI] - ↑
Roe, A.W., & Ts'o, D.Y. (1995).
Visual topography in primate V2: multiple representation across functional stripes. The Journal of neuroscience : the official journal of the Society for Neuroscience, 15(5 Pt 2), 3689-715. [PubMed:7751939] [WorldCat] - ↑
Shipp, S., & Zeki, S. (2002).
The functional organization of area V2, I: specialization across stripes and layers. Visual neuroscience, 19(2), 187-210. [PubMed:12385630] [WorldCat] [DOI] - ↑
Shipp, S., & Zeki, S. (2002).
The functional organization of area V2, II: the impact of stripes on visual topography. Visual neuroscience, 19(2), 211-31. [PubMed:12385631] [WorldCat] [DOI] - ↑
Livingstone, M., & Hubel, D. (1988).
Segregation of form, color, movement, and depth: anatomy, physiology, and perception. Science (New York, N.Y.), 240(4853), 740-9. [PubMed:3283936] [WorldCat] [DOI] - ↑
Sincich, L.C., & Horton, J.C. (2005).
The circuitry of V1 and V2: integration of color, form, and motion. Annual review of neuroscience, 28, 303-26. [PubMed:16022598] [WorldCat] [DOI] - ↑
Federer, F., Williams, D., Ichida, J.M., Merlin, S., & Angelucci, A. (2013).
Two projection streams from macaque V1 to the pale cytochrome oxidase stripes of V2. The Journal of neuroscience : the official journal of the Society for Neuroscience, 33(28), 11530-9. [PubMed:23843523] [PMC] [WorldCat] [DOI] - ↑
Thomas, O.M., Cumming, B.G., & Parker, A.J. (2002).
A specialization for relative disparity in V2. Nature neuroscience, 5(5), 472-8. [PubMed:11967544] [WorldCat] [DOI] - ↑
Hegdé, J., & Van Essen, D.C. (2000).
Selectivity for complex shapes in primate visual area V2. The Journal of neuroscience : the official journal of the Society for Neuroscience, 20(5), RC61. [PubMed:10684908] [PMC] [WorldCat] - ↑
Ito, M., & Komatsu, H. (2004).
Representation of angles embedded within contour stimuli in area V2 of macaque monkeys. The Journal of neuroscience : the official journal of the Society for Neuroscience, 24(13), 3313-24. [PubMed:15056711] [PMC] [WorldCat] [DOI] - ↑
Willmore, B.D., Prenger, R.J., & Gallant, J.L. (2010).
Neural representation of natural images in visual area V2. The Journal of neuroscience : the official journal of the Society for Neuroscience, 30(6), 2102-14. [PubMed:20147538] [PMC] [WorldCat] [DOI] - ↑ 100.0 100.1
Zeki, S.M. (1969).
Representation of central visual fields in prestriate cortex of monkey. Brain research, 14(2), 271-91. [PubMed:4978525] [WorldCat] [DOI] - ↑
Lyon, D.C., & Kaas, J.H. (2002).
Evidence for a modified V3 with dorsal and ventral halves in macaque monkeys. Neuron, 33(3), 453-61. [PubMed:11832231] [WorldCat] [DOI] - ↑
Gegenfurtner, K.R., Kiper, D.C., & Levitt, J.B. (1997).
Functional properties of neurons in macaque area V3. Journal of neurophysiology, 77(4), 1906-23. [PubMed:9114244] [WorldCat] [DOI] - ↑
Newsome, W.T., Maunsell, J.H., & Van Essen, D.C. (1986).
Ventral posterior visual area of the macaque: visual topography and areal boundaries. The Journal of comparative neurology, 252(2), 139-53. [PubMed:3782504] [WorldCat] [DOI] - ↑
Burkhalter, A., Felleman, D.J., Newsome, W.T., & Van Essen, D.C. (1986).
Anatomical and physiological asymmetries related to visual areas V3 and VP in macaque extrastriate cortex. Vision research, 26(1), 63-80. [PubMed:3716214] [WorldCat] [DOI] - ↑
De Weerd, P., Gattass, R., Desimone, R., & Ungerleider, L.G. (1995).
Responses of cells in monkey visual cortex during perceptual filling-in of an artificial scotoma. Nature, 377(6551), 731-4. [PubMed:7477262] [WorldCat] [DOI] - ↑
Nakamura, K., & Colby, C.L. (2000).
Visual, saccade-related, and cognitive activation of single neurons in monkey extrastriate area V3A. Journal of neurophysiology, 84(2), 677-92. [PubMed:10938295] [WorldCat] [DOI] - ↑
McKeefry, D.J., Burton, M.P., Vakrou, C., Barrett, B.T., & Morland, A.B. (2008).
Induced deficits in speed perception by transcranial magnetic stimulation of human cortical areas V5/MT+ and V3A. The Journal of neuroscience : the official journal of the Society for Neuroscience, 28(27), 6848-57. [PubMed:18596160] [PMC] [WorldCat] [DOI] - ↑
Clifford, C.W., Freedman, J.N., & Vaina, L.M. (1998).
First- and second-order motion perception in Gabor micropattern stimuli: psychophysics and computational modelling. Brain research. Cognitive brain research, 6(4), 263-71. [PubMed:9593930] [WorldCat] - ↑
Press, W.A., Brewer, A.A., Dougherty, R.F., Wade, A.R., & Wandell, B.A. (2001).
Visual areas and spatial summation in human visual cortex. Vision research, 41(10-11), 1321-32. [PubMed:11322977] [WorldCat] [DOI] - ↑ 110.0 110.1
Armendariz, M., Ban, H., Welchman, A.E., & Vanduffel, W. (2019).
Areal differences in depth cue integration between monkey and human. PLoS biology, 17(3), e2006405. [PubMed:30925163] [PMC] [WorldCat] [DOI] - ↑ 111.0 111.1
Hansen, K.A., Kay, K.N., & Gallant, J.L. (2007).
Topographic organization in and near human visual area V4. The Journal of neuroscience : the official journal of the Society for Neuroscience, 27(44), 11896-911. [PubMed:17978030] [PMC] [WorldCat] [DOI] - ↑
Nakamura, H., Gattass, R., Desimone, R., & Ungerleider, L.G. (1993).
The modular organization of projections from areas V1 and V2 to areas V4 and TEO in macaques. The Journal of neuroscience : the official journal of the Society for Neuroscience, 13(9), 3681-91. [PubMed:7690064] [PMC] [WorldCat] - ↑
Roe, A.W., Chelazzi, L., Connor, C.E., Conway, B.R., Fujita, I., Gallant, J.L., ..., & Vanduffel, W. (2012).
Toward a unified theory of visual area V4. Neuron, 74(1), 12-29. [PubMed:22500626] [PMC] [WorldCat] [DOI] - ↑
Pasupathy, A., Popovkina, D.V., & Kim, T. (2020).
Visual Functions of Primate Area V4. Annual review of vision science, 6, 363-385. [PubMed:32580663] [PMC] [WorldCat] [DOI] - ↑
Zeki, S.M. (1973).
Colour coding in rhesus monkey prestriate cortex. Brain research, 53(2), 422-7. [PubMed:4196224] [WorldCat] [DOI] - ↑
Essen, D.C., & Zeki, S.M. (1978).
The topographic organization of rhesus monkey prestriate cortex. The Journal of physiology, 277, 193-226. [PubMed:418173] [PMC] [WorldCat] [DOI] - ↑
Tanaka, M., Weber, H., & Creutzfeldt, O.D. (1986).
Visual properties and spatial distribution of neurones in the visual association area on the prelunate gyrus of the awake monkey. Experimental brain research, 65(1), 11-37. [PubMed:3803497] [WorldCat] [DOI] - ↑
Tanigawa, H., Lu, H.D., & Roe, A.W. (2010).
Functional organization for color and orientation in macaque V4. Nature neuroscience, 13(12), 1542-8. [PubMed:21076422] [PMC] [WorldCat] [DOI] - ↑
Conway, B.R., Moeller, S., & Tsao, D.Y. (2007).
Specialized color modules in macaque extrastriate cortex. Neuron, 56(3), 560-73. [PubMed:17988638] [WorldCat] [DOI] - ↑
Hegdé, J., & Van Essen, D.C. (2007).
A comparative study of shape representation in macaque visual areas v2 and v4. Cerebral cortex (New York, N.Y. : 1991), 17(5), 1100-16. [PubMed:16785255] [WorldCat] [DOI] - ↑
Pasupathy, A., & Connor, C.E. (1999).
Responses to contour features in macaque area V4. Journal of neurophysiology, 82(5), 2490-502. [PubMed:10561421] [WorldCat] [DOI] - ↑
Gallant, J.L., Braun, J., & Van Essen, D.C. (1993).
Selectivity for polar, hyperbolic, and Cartesian gratings in macaque visual cortex. Science (New York, N.Y.), 259(5091), 100-3. [PubMed:8418487] [WorldCat] [DOI] - ↑
Pasupathy, A., & Connor, C.E. (1999).
Responses to contour features in macaque area V4. Journal of neurophysiology, 82(5), 2490-502. [PubMed:10561421] [WorldCat] [DOI] - ↑
Hinkle, D.A., & Connor, C.E. (2005).
Quantitative characterization of disparity tuning in ventral pathway area V4. Journal of neurophysiology, 94(4), 2726-37. [PubMed:15987762] [WorldCat] [DOI] - ↑
Desimone, R., & Schein, S.J. (1987).
Visual properties of neurons in area V4 of the macaque: sensitivity to stimulus form. Journal of neurophysiology, 57(3), 835-68. [PubMed:3559704] [WorldCat] [DOI] - ↑
Hanazawa, A., & Komatsu, H. (2001).
Influence of the direction of elemental luminance gradients on the responses of V4 cells to textured surfaces. The Journal of neuroscience : the official journal of the Society for Neuroscience, 21(12), 4490-7. [PubMed:11404436] [PMC] [WorldCat] - ↑
El-Shamayleh, Y., & Pasupathy, A. (2016).
Contour Curvature As an Invariant Code for Objects in Visual Area V4. The Journal of neuroscience : the official journal of the Society for Neuroscience, 36(20), 5532-43. [PubMed:27194333] [PMC] [WorldCat] [DOI] - ↑
Mysore, S.G., Vogels, R., Raiguel, S.E., & Orban, G.A. (2006).
Processing of kinetic boundaries in macaque V4. Journal of neurophysiology, 95(3), 1864-80. [PubMed:16267116] [WorldCat] [DOI] - ↑
Gallant, J.L., Connor, C.E., Rakshit, S., Lewis, J.W., & Van Essen, D.C. (1996).
Neural responses to polar, hyperbolic, and Cartesian gratings in area V4 of the macaque monkey. Journal of neurophysiology, 76(4), 2718-39. [PubMed:8899641] [WorldCat] [DOI] - ↑
Walsh, V., Carden, D., Butler, S.R., & Kulikowski, J.J. (1993).
The effects of V4 lesions on the visual abilities of macaques: hue discrimination and colour constancy. Behavioural brain research, 53(1-2), 51-62. [PubMed:8466667] [WorldCat] [DOI] - ↑
Schiller, P.H. (1993).
The effects of V4 and middle temporal (MT) area lesions on visual performance in the rhesus monkey. Visual neuroscience, 10(4), 717-46. [PubMed:8338809] [WorldCat] [DOI] - ↑
De Weerd, P., Desimone, R., & Ungerleider, L.G. (1996).
Cue-dependent deficits in grating orientation discrimination after V4 lesions in macaques. Visual neuroscience, 13(3), 529-38. [PubMed:8782380] [WorldCat] [DOI] - ↑
De Weerd, P., Peralta, M.R., Desimone, R., & Ungerleider, L.G. (1999).
Loss of attentional stimulus selection after extrastriate cortical lesions in macaques. Nature neuroscience, 2(8), 753-8. [PubMed:10412066] [WorldCat] [DOI] - ↑ 134.0 134.1
Wade, A.R., Brewer, A.A., Rieger, J.W., & Wandell, B.A. (2002).
Functional measurements of human ventral occipital cortex: retinotopy and colour. Philosophical transactions of the Royal Society of London. Series B, Biological sciences, 357(1424), 963-73. [PubMed:12217168] [PMC] [WorldCat] [DOI] - ↑
Pearlman, A.L., Birch, J., & Meadows, J.C. (1979).
Cerebral color blindness: an acquired defect in hue discrimination. Annals of neurology, 5(3), 253-61. [PubMed:312619] [WorldCat] [DOI] - ↑
Hadjikhani, N., Liu, A.K., Dale, A.M., Cavanagh, P., & Tootell, R.B. (1998).
Retinotopy and color sensitivity in human visual cortical area V8. Nature neuroscience, 1(3), 235-41. [PubMed:10195149] [WorldCat] [DOI] - ↑
Tootell, R.B., & Hadjikhani, N. (2001).
Where is 'dorsal V4' in human visual cortex? Retinotopic, topographic and functional evidence. Cerebral cortex (New York, N.Y. : 1991), 11(4), 298-311. [PubMed:11278193] [WorldCat] [DOI] - ↑
Allman, J.M., & Kaas, J.H. (1971).
A representation of the visual field in the caudal third of the middle tempral gyrus of the owl monkey (Aotus trivirgatus). Brain research, 31(1), 85-105. [PubMed:4998922] [WorldCat] [DOI] - ↑ 139.0 139.1
Dubner, R., & Zeki, S.M. (1971).
Response properties and receptive fields of cells in an anatomically defined region of the superior temporal sulcus in the monkey. Brain research, 35(2), 528-32. [PubMed:5002708] [WorldCat] [DOI] - ↑
Tootell, R.B., & Taylor, J.B. (1995).
Anatomical evidence for MT and additional cortical visual areas in humans. Cerebral cortex (New York, N.Y. : 1991), 5(1), 39-55. [PubMed:7719129] [WorldCat] [DOI] - ↑
Deyoe, E.A., Hockfield, S., Garren, H., & Van Essen, D.C. (1990).
Antibody labeling of functional subdivisions in visual cortex: Cat-301 immunoreactivity in striate and extrastriate cortex of the macaque monkey. Visual neuroscience, 5(1), 67-81. [PubMed:1702988] [WorldCat] [DOI] - ↑
Tootell, R.B., Reppas, J.B., Kwong, K.K., Malach, R., Born, R.T., Brady, T.J., ..., & Belliveau, J.W. (1995).
Functional analysis of human MT and related visual cortical areas using magnetic resonance imaging. The Journal of neuroscience : the official journal of the Society for Neuroscience, 15(4), 3215-30. [PubMed:7722658] [WorldCat] - ↑
Watson, J.D., Myers, R., Frackowiak, R.S., Hajnal, J.V., Woods, R.P., Mazziotta, J.C., ..., & Zeki, S. (1993).
Area V5 of the human brain: evidence from a combined study using positron emission tomography and magnetic resonance imaging. Cerebral cortex (New York, N.Y. : 1991), 3(2), 79-94. [PubMed:8490322] [WorldCat] [DOI] - ↑
Ungerleider, L.G., & Desimone, R. (1986).
Cortical connections of visual area MT in the macaque. The Journal of comparative neurology, 248(2), 190-222. [PubMed:3722458] [WorldCat] [DOI] - ↑
Palmer, S.M., & Rosa, M.G. (2006).
A distinct anatomical network of cortical areas for analysis of motion in far peripheral vision. The European journal of neuroscience, 24(8), 2389-405. [PubMed:17042793] [WorldCat] [DOI] - ↑
Sincich, L.C., Park, K.F., Wohlgemuth, M.J., & Horton, J.C. (2004).
Bypassing V1: a direct geniculate input to area MT. Nature neuroscience, 7(10), 1123-8. [PubMed:15378066] [WorldCat] [DOI] - ↑
Maunsell, J.H., & Van Essen, D.C. (1983).
Functional properties of neurons in middle temporal visual area of the macaque monkey. I. Selectivity for stimulus direction, speed, and orientation. Journal of neurophysiology, 49(5), 1127-47. [PubMed:6864242] [WorldCat] [DOI] - ↑
Felleman, D.J., & Kaas, J.H. (1984).
Receptive-field properties of neurons in middle temporal visual area (MT) of owl monkeys. Journal of neurophysiology, 52(3), 488-513. [PubMed:6481441] [WorldCat] [DOI] - ↑
Albright, T.D., Desimone, R., & Gross, C.G. (1984).
Columnar organization of directionally selective cells in visual area MT of the macaque. Journal of neurophysiology, 51(1), 16-31. [PubMed:6693933] [WorldCat] [DOI] - ↑
DeAngelis, G.C., & Newsome, W.T. (1999).
Organization of disparity-selective neurons in macaque area MT. The Journal of neuroscience : the official journal of the Society for Neuroscience, 19(4), 1398-415. [PubMed:9952417] [PMC] [WorldCat] - ↑
Czuba, T.B., Huk, A.C., Cormack, L.K., & Kohn, A. (2014).
Area MT encodes three-dimensional motion. The Journal of neuroscience : the official journal of the Society for Neuroscience, 34(47), 15522-33. [PubMed:25411482] [PMC] [WorldCat] [DOI] - ↑
Sanada, T.M., & DeAngelis, G.C. (2014).
Neural representation of motion-in-depth in area MT. The Journal of neuroscience : the official journal of the Society for Neuroscience, 34(47), 15508-21. [PubMed:25411481] [PMC] [WorldCat] [DOI] - ↑
Zihl, J., von Cramon, D., & Mai, N. (1983).
Selective disturbance of movement vision after bilateral brain damage. Brain : a journal of neurology, 106 (Pt 2), 313-40. [PubMed:6850272] [WorldCat] [DOI] - ↑
Hess, R.H., Baker, C.L., & Zihl, J. (1989).
The "motion-blind" patient: low-level spatial and temporal filters. The Journal of neuroscience : the official journal of the Society for Neuroscience, 9(5), 1628-40. [PubMed:2723744] [WorldCat] - ↑
Baker, C.L., Hess, R.F., & Zihl, J. (1991).
Residual motion perception in a "motion-blind" patient, assessed with limited-lifetime random dot stimuli. The Journal of neuroscience : the official journal of the Society for Neuroscience, 11(2), 454-61. [PubMed:1992012] [WorldCat] - ↑
Walsh, V., Ellison, A., Battelli, L., & Cowey, A. (1998).
Task-specific impairments and enhancements induced by magnetic stimulation of human visual area V5. Proceedings. Biological sciences, 265(1395), 537-43. [PubMed:9569672] [PMC] [WorldCat] [DOI] - ↑
Colby, C.L., Duhamel, J.R., & Goldberg, M.E. (1993).
Ventral intraparietal area of the macaque: anatomic location and visual response properties. Journal of neurophysiology, 69(3), 902-14. [PubMed:8385201] [WorldCat] [DOI] - ↑
Neuenschwander, S., Gattass, R., Sousa, A.P., & Piñon, M.C. (1994).
Identification and visuotopic organization of areas PO and POd in Cebus monkey. The Journal of comparative neurology, 340(1), 65-86. [PubMed:8176003] [WorldCat] [DOI] - ↑
Galletti, C., Gamberini, M., Kutz, D.F., Baldinotti, I., & Fattori, P. (2005).
The relationship between V6 and PO in macaque extrastriate cortex. The European journal of neuroscience, 21(4), 959-70. [PubMed:15787702] [WorldCat] [DOI] - ↑
Galletti, C., Fattori, P., Battaglini, P.P., Shipp, S., & Zeki, S. (1996).
Functional demarcation of a border between areas V6 and V6A in the superior parietal gyrus of the macaque monkey. The European journal of neuroscience, 8(1), 30-52. [PubMed:8713448] [WorldCat] [DOI] - ↑
Galletti, C., Fattori, P., Gamberini, M., & Kutz, D.F. (1999).
The cortical visual area V6: brain location and visual topography. The European journal of neuroscience, 11(11), 3922-36. [PubMed:10583481] [WorldCat] [DOI] - ↑
Shipp, S., Blanton, M., & Zeki, S. (1998).
A visuo-somatomotor pathway through superior parietal cortex in the macaque monkey: cortical connections of areas V6 and V6A. The European journal of neuroscience, 10(10), 3171-93. [PubMed:9786211] [WorldCat] [DOI] - ↑
Rosa, M.G., Palmer, S.M., Gamberini, M., Tweedale, R., Piñon, M.C., & Bourne, J.A. (2005).
Resolving the organization of the New World monkey third visual complex: the dorsal extrastriate cortex of the marmoset (Callithrix jacchus). The Journal of comparative neurology, 483(2), 164-91. [PubMed:15678474] [WorldCat] [DOI] - ↑ 164.0 164.1
Pitzalis, S., Galletti, C., Huang, R.S., Patria, F., Committeri, G., Galati, G., ..., & Sereno, M.I. (2006).
Wide-field retinotopy defines human cortical visual area v6. The Journal of neuroscience : the official journal of the Society for Neuroscience, 26(30), 7962-73. [PubMed:16870741] [PMC] [WorldCat] [DOI] - ↑
Galletti, C., Gamberini, M., Kutz, D.F., Fattori, P., Luppino, G., & Matelli, M. (2001).
The cortical connections of area V6: an occipito-parietal network processing visual information. The European journal of neuroscience, 13(8), 1572-88. [PubMed:11328351] [WorldCat] [DOI] - ↑
Pitzalis, S., Sereno, M.I., Committeri, G., Fattori, P., Galati, G., Patria, F., & Galletti, C. (2010).
Human v6: the medial motion area. Cerebral cortex (New York, N.Y. : 1991), 20(2), 411-24. [PubMed:19502476] [PMC] [WorldCat] [DOI] - ↑
Cardin, V., & Smith, A.T. (2011).
Sensitivity of human visual cortical area V6 to stereoscopic depth gradients associated with self-motion. Journal of neurophysiology, 106(3), 1240-9. [PubMed:21653717] [PMC] [WorldCat] [DOI] - ↑
Blanke, O., Landis, T., Mermoud, C., Spinelli, L., & Safran, A.B. (2003).
Direction-selective motion blindness after unilateral posterior brain damage. The European journal of neuroscience, 18(3), 709-22. [PubMed:12911768] [WorldCat] [DOI]