滑面小胞体
大久保 洋平
順天堂大学医学部薬理学講座
DOI:10.14931/bsd.504 原稿受付日:2022年9月30日 原稿完成日:2022年10月12日
担当編集委員:和田 圭司(国立研究開発法人国立精神・神経医療研究センター)
英: smooth endoplasmic reticulum 独:glattes endoplasmatisches Retikulum, agranuläres endoplasmatisches Retikulum 仏:réticulum endoplasmique lisse
英略号:smooth ER, SER
細胞内小器官である小胞体のうち、リボソームが膜上に存在する粗面小胞体ではない部分を滑面小胞体と総称する。タンパク質輸送、脂質合成、物質代謝、オートファゴソーム生成などの多様な機能を担うとともに、細胞内カルシウムストアとして機能する。
滑面小胞体とは
小胞体は脂質二重膜に囲まれた細胞内小器官であり、粗面小胞体と滑面小胞体からなる。粗面小胞体は多数のリボソームが膜上に存在する外観から名付けられ、膜タンパク質および分泌タンパク質の合成を主な機能とする。一方、滑面小胞体は粗面小胞体ではない部分の総称であり、タンパク質輸送、脂質合成、物質代謝、オートファゴソーム生成などの多様な機能を担うとともに、細胞内カルシウムストアとして機能する。
構造
神経細胞において滑面小胞体は直径40〜80 nm程度の細管状形態を基本要素とし、細管が分岐・融合することで網状の形態を示す。また一部では扁平嚢状の形態を示し、細胞膜直下に形成されるsubsurface cisternやスパイン内部に形成されるspine apparatus等の神経細胞に特徴的な形態が存在する[2]。スパイン、樹状突起、細胞体、軸索、シナプス前終末に至るまで、細胞全体に張り巡らされた一繋がりのネットワークを形成し[3]、各所で多様な機能を果たすと考えられる。スパイン内滑面小胞体については、海馬CA1錐体細胞では一部のスパインにしか存在しないが[4]、小脳プルキンエ細胞ではほぼ全てのスパインに存在する[5]という多様性も見られる(図1)。
一方、粗面小胞体は細胞体とその周辺に分布が限られている。
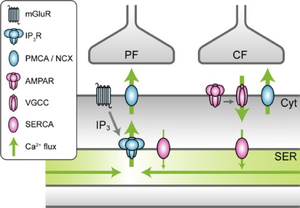
プルキンエ細胞へのシナプス入力に伴い惹起されるCa2+の流れ。mGluR: 代謝型グルタミン酸受容体、IP3R: イノシトール三リン酸受容体、PMCA/NCX: 細胞膜Ca2+ ATPアーゼ/Na+-Ca2+交換体、AMPAR: AMPA型グルタミン酸受容体、VGCC: 電位依存性Ca2+チャネル、SERCA: 筋小胞体/小胞体Ca2+ ATPアーゼ、Ca2+ flux: Ca2+の流れ、PF: 平行線維、CF: 登上線維、Cyt: 細胞質、SER: 滑面小胞体。文献[1]より改変。
機能
細胞内カルシウムストア
滑面小胞体はCa2+取り込みおよびCa2+放出機構を有し、様々な細胞で細胞内カルシウムストアとして中心的な役割を担っている(詳細は細胞内カルシウムストアを参照)。神経細胞においても滑面小胞体は細胞内カルシウムストアとして機能し、多様なCa2+依存性シグナルに関与する[6]。
特筆すべき機能としては、シナプス前部および後部における局所的なCa2+動態の形成を通じて、シナプス機能を制御することが挙げられる(図2)。シナプス前終末において、リアノジン受容体を介した小胞体からのCa2+放出は自発的および活動依存的伝達物質放出を亢進させる[7][8][9][10][11]、ただし[12]も参照)。また小胞体のCa2+取込能力や内腔Ca2+量の低下はシナプス小胞の放出やリサイクリングを阻害する[13][14]。
シナプス後部のスパインにおいては、NMDA型グルタミン酸受容体を介したリアノジン受容体の活性化[15]および代謝型グルタミン酸受容体を介したイノシトール三リン酸受容体の活性化 [16][17] により、単一スパインに限局した小胞体Ca2+放出が惹起され、これらは各種のシナプス可塑性の誘導に関与すると考えられる[18]。一例として、スパインにおける滑面小胞体の有無と、代謝型グルタミン酸受容体依存性長期抑圧の有無が相関することが報告されている[19]。
また後シナプスにおける代謝型グルタミン酸受容体の活性化が一定の強度を超えると、イノシトール三リン酸受容体を介した小胞体Ca2+放出が樹状突起に沿って連鎖的に伝播することで、Ca2+ウェーブが惹起される[20]。入力を受けたシナプスの周囲におけるヘテロシナプス可塑性に関与する可能性も指摘されているが[21]、神経細胞におけるCa2+ウェーブの機能的意義は現在のところ不明瞭である[22]。
さらに滑面小胞体はその内腔をCa2+が拡散・移動することで、効率的な細胞内Ca2+輸送系として機能する[23]。樹状突起におけるCa2+放出の後に滑面小胞体Ca2+は局所的に枯渇するが、滑面小胞体ネットワークを通じたCa2+拡散により速やかに再充填される[24][1](図3)。
膜タンパク質輸送
神経細胞の細胞体においては他の細胞同様、粗面小胞体近傍に存在するER exit siteからゴルジ体への小胞輸送により膜タンパク質が輸送される。そしてゴルジ体から分泌された小胞は、細胞内輸送系により樹状突起および軸索先端まで届けられる。しかし神経細胞は長大な突起を有し、さらに活動依存的に突起局所の膜タンパク質発現量が調節され得るため、ゴルジ体を介した通常の経路だけでは効率的な制御が困難であると考えられる。
実際にもう一つのメカニズムとして、細胞体から離れた樹状突起において、膜タンパク質の局所タンパク質合成が行われることが報告されている。樹状突起にはゴルジ体様の小器官であるGolgi outpostが存在し、近傍の滑面小胞体からの小胞輸送を受けて、翻訳された膜タンパク質を樹状突起局所の細胞膜に輸送する[25]。またGolgi outpostを経ない局所分泌経路の存在も示唆されている[25]。さらに翻訳された膜タンパク質が滑面小胞体の膜上を拡散することが、樹状突起に沿った膜タンパク質輸送機構として機能し得ることも報告されている[26]。
疾患との関わり
小胞体形態形成に関わるタンパク質をコードする遺伝子の変異と、筋萎縮性側索硬化症および遺伝性痙性対麻痺との関連が報告されており[27][28][29][30][31] 、滑面小胞体ネットワーク構造の神経細胞機能への重要性が示唆される。また滑面小胞体Ca2+恒常性に関わる機構の変調と、各種神経変性疾患との関係が報告されており[32]、正常な細胞内カルシウムストア機能が神経細胞の生存に不可欠であることが示唆される。
関連項目
参考文献
- ↑ 以下の位置に戻る: 1.0 1.1 1.2
Okubo, Y., Suzuki, J., Kanemaru, K., Nakamura, N., Shibata, T., & Iino, M. (2015).
Visualization of Ca2+ Filling Mechanisms upon Synaptic Inputs in the Endoplasmic Reticulum of Cerebellar Purkinje Cells. The Journal of neuroscience : the official journal of the Society for Neuroscience, 35(48), 15837-46. [PubMed:26631466] [PMC] [WorldCat] [DOI] - ↑
Wu, Y., Whiteus, C., Xu, C.S., Hayworth, K.J., Weinberg, R.J., Hess, H.F., & De Camilli, P. (2017).
Contacts between the endoplasmic reticulum and other membranes in neurons. Proceedings of the National Academy of Sciences of the United States of America, 114(24), E4859-E4867. [PubMed:28559323] [PMC] [WorldCat] [DOI] - ↑
Terasaki, M., Slater, N.T., Fein, A., Schmidek, A., & Reese, T.S. (1994).
Continuous network of endoplasmic reticulum in cerebellar Purkinje neurons. Proceedings of the National Academy of Sciences of the United States of America, 91(16), 7510-4. [PubMed:7519781] [PMC] [WorldCat] [DOI] - ↑
Spacek, J., & Harris, K.M. (1997).
Three-dimensional organization of smooth endoplasmic reticulum in hippocampal CA1 dendrites and dendritic spines of the immature and mature rat. The Journal of neuroscience : the official journal of the Society for Neuroscience, 17(1), 190-203. [PubMed:8987748] [PMC] [WorldCat] - ↑
Harris, K.M., & Stevens, J.K. (1988).
Dendritic spines of rat cerebellar Purkinje cells: serial electron microscopy with reference to their biophysical characteristics. The Journal of neuroscience : the official journal of the Society for Neuroscience, 8(12), 4455-69. [PubMed:3199186] [PMC] [WorldCat] - ↑
Berridge, M.J. (1998).
Neuronal calcium signaling. Neuron, 21(1), 13-26. [PubMed:9697848] [WorldCat] [DOI] - ↑
Llano, I., González, J., Caputo, C., Lai, F.A., Blayney, L.M., Tan, Y.P., & Marty, A. (2000).
Presynaptic calcium stores underlie large-amplitude miniature IPSCs and spontaneous calcium transients. Nature neuroscience, 3(12), 1256-65. [PubMed:11100146] [WorldCat] [DOI] - ↑
Emptage, N.J., Reid, C.A., & Fine, A. (2001).
Calcium stores in hippocampal synaptic boutons mediate short-term plasticity, store-operated Ca2+ entry, and spontaneous transmitter release. Neuron, 29(1), 197-208. [PubMed:11182091] [WorldCat] [DOI] - ↑
Sharma, G., & Vijayaraghavan, S. (2003).
Modulation of presynaptic store calcium induces release of glutamate and postsynaptic firing. Neuron, 38(6), 929-39. [PubMed:12818178] [WorldCat] [DOI] - ↑
Galante, M., & Marty, A. (2003).
Presynaptic ryanodine-sensitive calcium stores contribute to evoked neurotransmitter release at the basket cell-Purkinje cell synapse. The Journal of neuroscience : the official journal of the Society for Neuroscience, 23(35), 11229-34. [PubMed:14657182] [PMC] [WorldCat] - ↑
Shimizu, H., Fukaya, M., Yamasaki, M., Watanabe, M., Manabe, T., & Kamiya, H. (2008).
Use-dependent amplification of presynaptic Ca2+ signaling by axonal ryanodine receptors at the hippocampal mossy fiber synapse. Proceedings of the National Academy of Sciences of the United States of America, 105(33), 11998-2003. [PubMed:18687898] [PMC] [WorldCat] [DOI] - ↑
Carter, A.G., Vogt, K.E., Foster, K.A., & Regehr, W.G. (2002).
Assessing the role of calcium-induced calcium release in short-term presynaptic plasticity at excitatory central synapses. The Journal of neuroscience : the official journal of the Society for Neuroscience, 22(1), 21-8. [PubMed:11756484] [PMC] [WorldCat] - ↑
de Juan-Sanz, J., Holt, G.T., Schreiter, E.R., de Juan, F., Kim, D.S., & Ryan, T.A. (2017).
Axonal Endoplasmic Reticulum Ca2+ Content Controls Release Probability in CNS Nerve Terminals. Neuron, 93(4), 867-881.e6. [PubMed:28162809] [PMC] [WorldCat] [DOI] - ↑
Lindhout, F.W., Cao, Y., Kevenaar, J.T., Bodzęta, A., Stucchi, R., Boumpoutsari, M.M., ..., & Hoogenraad, C.C. (2019).
VAP-SCRN1 interaction regulates dynamic endoplasmic reticulum remodeling and presynaptic function. The EMBO journal, 38(20), e101345. [PubMed:31441084] [PMC] [WorldCat] [DOI] - ↑
Emptage, N., Bliss, T.V., & Fine, A. (1999).
Single synaptic events evoke NMDA receptor-mediated release of calcium from internal stores in hippocampal dendritic spines. Neuron, 22(1), 115-24. [PubMed:10027294] [WorldCat] [DOI] - ↑
Finch, E.A., & Augustine, G.J. (1999).
Local calcium signalling by inositol-1,4,5-trisphosphate in Purkinje cell dendrites. Nature, 396(6713), 753-6. [PubMed:9874372] [WorldCat] [DOI] - ↑
Takechi, H., Eilers, J., & Konnerth, A. (1999).
A new class of synaptic response involving calcium release in dendritic spines. Nature, 396(6713), 757-60. [PubMed:9874373] [WorldCat] [DOI] - ↑
Padamsey, Z., Foster, W.J., & Emptage, N.J. (2019).
Intracellular Ca2+ Release and Synaptic Plasticity: A Tale of Many Stores. The Neuroscientist : a review journal bringing neurobiology, neurology and psychiatry, 25(3), 208-226. [PubMed:30014771] [WorldCat] [DOI] - ↑
Holbro, N., Grunditz, A., & Oertner, T.G. (2009).
Differential distribution of endoplasmic reticulum controls metabotropic signaling and plasticity at hippocampal synapses. Proceedings of the National Academy of Sciences of the United States of America, 106(35), 15055-60. [PubMed:19706463] [PMC] [WorldCat] [DOI] - ↑
Nakamura, T., Barbara, J.G., Nakamura, K., & Ross, W.N. (1999).
Synergistic release of Ca2+ from IP3-sensitive stores evoked by synaptic activation of mGluRs paired with backpropagating action potentials. Neuron, 24(3), 727-37. [PubMed:10595522] [WorldCat] [DOI] - ↑
Nishiyama, M., Hong, K., Mikoshiba, K., Poo, M.M., & Kato, K. (2000).
Calcium stores regulate the polarity and input specificity of synaptic modification. Nature, 408(6812), 584-8. [PubMed:11117745] [WorldCat] [DOI] - ↑
Ross, W.N. (2012).
Understanding calcium waves and sparks in central neurons. Nature reviews. Neuroscience, 13(3), 157-68. [PubMed:22314443] [PMC] [WorldCat] [DOI] - ↑
Mogami, H., Nakano, K., Tepikin, A.V., & Petersen, O.H. (1997).
Ca2+ flow via tunnels in polarized cells: recharging of apical Ca2+ stores by focal Ca2+ entry through basal membrane patch. Cell, 88(1), 49-55. [PubMed:9019404] [WorldCat] [DOI] - ↑
Choi, Y.M., Kim, S.H., Chung, S., Uhm, D.Y., & Park, M.K. (2006).
Regional interaction of endoplasmic reticulum Ca2+ signals between soma and dendrites through rapid luminal Ca2+ diffusion. The Journal of neuroscience : the official journal of the Society for Neuroscience, 26(47), 12127-36. [PubMed:17122037] [PMC] [WorldCat] [DOI] - ↑ 以下の位置に戻る: 25.0 25.1
Horton, A.C., & Ehlers, M.D. (2003).
Dual modes of endoplasmic reticulum-to-Golgi transport in dendrites revealed by live-cell imaging. The Journal of neuroscience : the official journal of the Society for Neuroscience, 23(15), 6188-99. [PubMed:12867502] [PMC] [WorldCat] - ↑
Cui-Wang, T., Hanus, C., Cui, T., Helton, T., Bourne, J., Watson, D., ..., & Ehlers, M.D. (2012).
Local zones of endoplasmic reticulum complexity confine cargo in neuronal dendrites. Cell, 148(1-2), 309-21. [PubMed:22265418] [PMC] [WorldCat] [DOI] - ↑
Yang, Y.S., Harel, N.Y., & Strittmatter, S.M. (2009).
Reticulon-4A (Nogo-A) redistributes protein disulfide isomerase to protect mice from SOD1-dependent amyotrophic lateral sclerosis. The Journal of neuroscience : the official journal of the Society for Neuroscience, 29(44), 13850-9. [PubMed:19889996] [PMC] [WorldCat] [DOI] - ↑
Fasana, E., Fossati, M., Ruggiano, A., Brambillasca, S., Hoogenraad, C.C., Navone, F., ..., & Borgese, N. (2010).
A VAPB mutant linked to amyotrophic lateral sclerosis generates a novel form of organized smooth endoplasmic reticulum. FASEB journal : official publication of the Federation of American Societies for Experimental Biology, 24(5), 1419-30. [PubMed:20008544] [WorldCat] [DOI] - ↑
Blackstone, C., O'Kane, C.J., & Reid, E. (2011).
Hereditary spastic paraplegias: membrane traffic and the motor pathway. Nature reviews. Neuroscience, 12(1), 31-42. [PubMed:21139634] [PMC] [WorldCat] [DOI] - ↑
Montenegro, G., Rebelo, A.P., Connell, J., Allison, R., Babalini, C., D'Aloia, M., ..., & Züchner, S. (2012).
Mutations in the ER-shaping protein reticulon 2 cause the axon-degenerative disorder hereditary spastic paraplegia type 12. The Journal of clinical investigation, 122(2), 538-44. [PubMed:22232211] [PMC] [WorldCat] [DOI] - ↑
Yalçın, B., Zhao, L., Stofanko, M., O'Sullivan, N.C., Kang, Z.H., Roost, A., ..., & O'Kane, C.J. (2017).
Modeling of axonal endoplasmic reticulum network by spastic paraplegia proteins. eLife, 6. [PubMed:28742022] [PMC] [WorldCat] [DOI] - ↑
Okubo, Y., Mikami, Y., Kanemaru, K., & Iino, M. (2018).
Role of Endoplasmic Reticulum-Mediated Ca2+ Signaling in Neuronal Cell Death. Antioxidants & redox signaling, 29(12), 1147-1157. [PubMed:29361832] [WorldCat] [DOI]